J. Craig Venter |
 |
|
 |
 |
 |

|

 |
J. Craig Venter |
|
Celera Genomics |
|
 |
 |
|
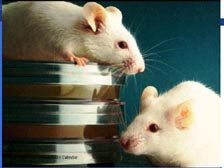
[figure 30]
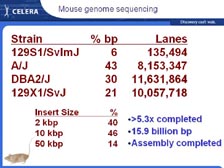
[figure 31]
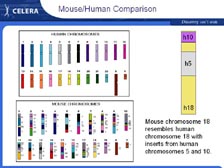
[figure 32]
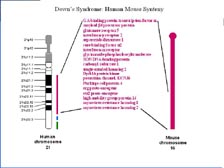
[figure 33]
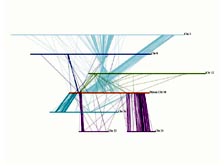
[figure 34]
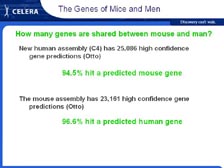
[figure 35]
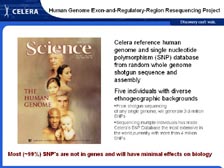
[figure 36]
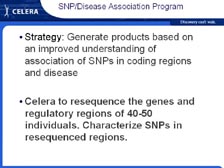
[figure 37]
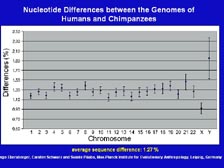
[figure 38]
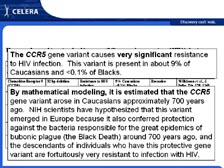
[figure 39]
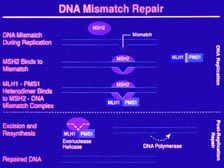
[figure 40]
|
[Figure 30] As soon as we finished sequencing the human genome, we sequenced the mouse genome, because we knew we would need the mouse to compare and understand the human. [Figure 31] We chose four different strains, and it took only six months to sequence the mouse genome. That's been assembled and that's been annotated, and Celera database users have been using this now for some time. [Figure 32] Most scientists in this field know that human chromosomes are just slightly different package rearrangements of large blocks of material from the mouse chromosomes. [Figure 33] If we zoom in to Human Chromosome 21, where so much work was done in Japan, we find in the Down's Syndrome region that all the genes are in the same exact order on Human Chromosome 21 as we find on the tip of Mouse Chromosome 16. Most people don't realize individuals with Down's Syndrome usually die by age 35. However, almost 100 percent of the ones that live beyond age 35 get Alzheimer's disease. Therefore, there was a lot of excitement within the scientific community when the second gene on this list, the Amyloid Precursor Protein, was found in this region. It has helped develop a mouse model of Alzheimer's disease, and it has also helped us understand why these individuals over 35 get Alzheimer's disease.
[Figure 34] The real situation is much more complicated. This is the first picture of what really happened in the evolution between mouse and humans in the last 60 million years. In the bottom right, the purple area is the duplication I just showed you on the Down's Syndrome region. You can see they came from the end of Mouse Chromosome 16. However, you can see the other human chromosomes all derived large blocks of genes in the same exact order from parts of Mouse Chromosome 16. Occasionally there are individual genes that moved around, that we don't yet know how to explain, but it's clear now that these large blocks move around. In every mammal we will look at, we will find these same genes in the same order.
[Figure 35] Therefore, one of the key questions about the genes of mice and men is: How many genes are shared in an exact fashion? If we look at 25,000 high confidence genes in the human genome, we find almost 95 percent of these hit a corresponding gene in the mouse genome. If we look at 23,000 genes in the mouse genome, we will find almost 97 percent of those precisely hit the human gene. These numbers aren't finished yet, and they will never match up exactly because in the last 60 million years there were still lots of duplication events that took place in a tandem fashion down the chromosomes. Nevertheless, this shows you how closely we are related at the gene level to other mammals.
[Figure 36] When we sequence the human genome with the whole genome shotgun method, we got around 4 million single nucleotide polymorphisms (SNPs), but when we looked at one individual's genome that we had most of the coverage on, we found only 2,000 SNPs (i.e., 2,000 letters in the genetic code that actually resulted in the change in the protein sequence wherever in a regulatory region). That says to me that probably 99 percent of all the variation in our genetic code plays little or no biological role. They are important, as Mike said, as forensic tools for personal identification and as mapping tools to help geneticists, but if we want to understand biological changes we need to understand the variance in genes and regulatory regions.
[Figure 37] As Mike said, we are now in the process of re-sequencing the genomes of 50 individuals, including all the genes and 5 KB upstream of the regulatory regions from these 50 individuals and two chimpanzees. This process will be completed in about one year, and at that time we will have the new tools to sequence all the genes and any one of your genomes in less than one week. Look at the change in the short period of time from the genome project being a 15 to 20 year, multi-billion dollar public project to, with the new tools developed by Applied Biosystems and others, only nine months at Celera, to within two years when we will be able to get the essential information out of the genome in less than one week. I don't think it's a great extrapolation to think that in five years, maybe ten years, if we look at this same rate of growth, that just during the course of this lecture, probably for a few thousand dollars, each of you could have your genomes completely sequenced. That's going to have a profound impact on how we study human biology, human variation and how we practice medicine.
[Figure 38] We have been asked many times why we do not sequence the chimpanzee genome, and I have argued that sequencing the chimpanzee genome is the equivalent to sequencing another human genome with just a slightly higher polymorphism rate. Svante Paabo, at the Max Planck Institute for Evolutionary Anthropology, sequenced a large number of sequences from the chimpanzee. He compared them to the Celera database and found, on average, only 1.27 percent difference across the entire genome. If you look down, here, at the X-chromosome that women have two of and men have one of, it varies only 0.9 percent between humans and chimpanzees. That includes the 75 percent of the chromosome that's just intragenic DNA, the 24 percent of the chromosome that's intron, and the 1.1 percent that actually codes for proteins of regulatory regions. The Y-chromosome, that is unique to males, can apparently vary quite dramatically between humans and chimps with very similar behavioral outcomes. Some of these variations can play an important role in biology, but not in the way that most people think. There has been a lot of talk about genetic determinism. That's why some scientists and people in the public wanted to have a large number of genes so there would be a gene for every human trait, every human condition. There is not. We have the same genes as chimpanzees, as mice, as rats, as dogs and cats. We regulate them differently, and minor variations in these genes can help lead to disease, but in most cases do not in an absolute sense.
[Figure 39] There are some changes that make us more susceptible to some diseases and some changes that make us less susceptible. With HIV there are a number of polymorphisms (changes in individual genes) that delay the onset of AIDS symptoms. As you can see from the bottom, there are a few gene changes that lead to very rapid HIV infection and early death. One of the genes that causes very significant resistance to HIV is very interesting. It's a variant in the CCR5 gene. It is present in about 9 percent of Caucasians, but only one/tenth of one percent of Blacks. What does that tell us? It tells us it was a very recent event in evolution. We can trace back that event to about 700 years ago. It turns out this same variation in the CCR5 gene gives resistance to Yersinia pestis infection, the agent that causes plague. So, if you are resistant to the plague, you would have survived, while millions of people throughout Europe were killed from this disease. It's easy to understand the population genetics of how a variant in the population, that was probably a minor variant originally, increased to 9 percent of the population. At the same time, because the plague didn't have the same impact in Africa, there was no selective pressure for that trait to increase.
[Figure 40] Early on we found three new genes that Burt Vogelstein, at Johns Hopkins University, linked to colon cancer. These were the mismatch DNA repair enzymes. There is now a clinical test that will measure your DNA repair enzymes and tell you whether you have an increased risk of getting colon cancer, but they won't tell you definitely if you will get colon cancer. If you don't have the changes, that won't tell you that you will be immune from colon cancer. In fact, early on I was puzzled. Why do you get colon cancer if these enzymes are deficient in every one of our hundred trillion cells? Why not brain cancer? Why not liver cancer? In fact, it's because colon cancer is not a genetic disease. It's an environmental disease that's caused by toxins in the colon, toxins from bacteria metabolites, toxins from what we eat that damage the DNA in the colon epithelial cells. If you get sufficient damage, those cells will become cancerous. If you can't repair that DNA damage very effectively, your chance of getting colon cancer goes up. So, is that a genetic disease or is that an environmental disease? It is both. I think people looking for exact links and exact causality will be very disappointed as we move into this new world of probabilities.
|
|
|